A custom-built microscope is giving scientists the closest view yet of living nerve synapses.
The brain hosts an extraordinarily complex network of interconnected nerve cells that are constantly exchanging electrical and chemical signals at speeds difficult to comprehend.
Understanding the detailed workings of a synapse—the junction between neurons that govern how these cells communicate with each other—is vital for modeling brain networks and understanding how diseases such as depression, Alzheimer’s, or schizophrenia may affect brain function, according to the researchers.
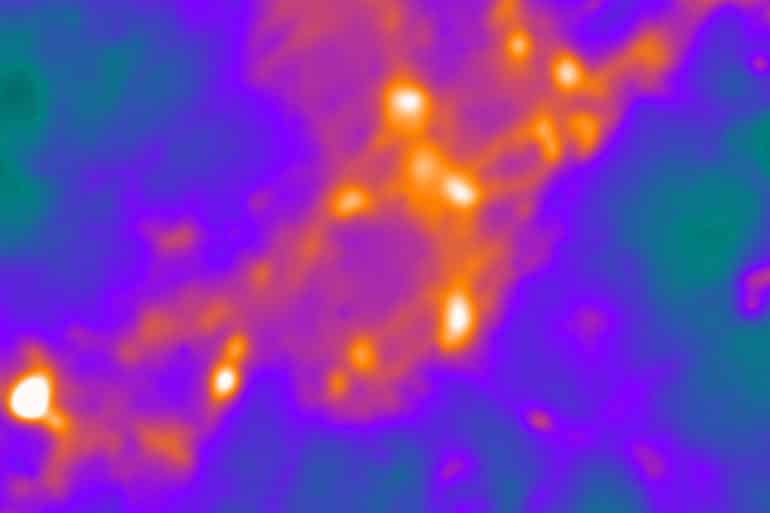
Studying active rat neurons, even those growing in a dish, is a challenge because they are so small. Further, they move, making it difficult to keep them in focus at high magnifications under a light microscope.
“Synapses are little nanoscale machines that transmit information,” says senior author Vitaly A. Klyachko, associate professor of cell biology and physiology at Washington University in St. Louis School of Medicine. “They’re very difficult to study because their scale is below what conventional light microscopes can resolve. So what is happening in the active zone of a synapse looks like a blur.
Artificial synapse could make computers more like brains
“To remedy this, our custom-built microscope has a very sensitive camera and is extremely stable at body temperatures, but most of the novelty comes from the analysis of the images,” he says. “Our approach gives us the ability to resolve events in the synapse with high precision.”
Until now, close-up views of the active zone have been provided by electron microscopes. While offering resolutions of mere tens of nanometers—about 1,000 times thinner than a human hair and smaller—electron microscopes can’t view living cells. To withstand bombardment by electrons, samples must be fixed in an epoxy resin or flash frozen, cut into extremely thin slices and coated in a layer of metal atoms.
“Most of what we know about the active zone is from indirect studies, including beautiful electron microscopy images,” says Klyachko, also an associate professor of biomedical engineering at the School of Engineering and Applied Science. “But these are static pictures. We wanted to develop a way to see the synapse function.”
A synapse consists of a tiny gap between two nerves, with one nerve serving as the transmitter and the other as the receiver. When sending signals, the transmitting side of the synapse releases little packages of neurotransmitters, which traverse the gap and bind to receptors on the receiving side, completing the information relay. On the transmitting side of the synapse the neurotransmitters at the active zone are packaged into synaptic vesicles.
“One of the most fundamental questions is: Are there many places at the active zone where a vesicle can release its neurotransmitters into the gap, or is there only one?” Klyachko says. “A lot of indirect measurements suggested there might be only one, or maybe two to three, at most.”
Neurons never quite recover from ‘Pavlov’s bell’
In other words, if the active zone could be compared to a shower head, the question would be whether it functions more as a single jet or as a rain shower.
The findings of the new study, published in the journal Neuron, show the active zone is more of a rain shower. But it’s not a random shower; there are about 10 locations dotted across the active zone that are reused too often to be left to chance. There is also a limit to how quickly these sites can be reused—about 100 milliseconds must pass before an individual site can be used again. And at higher rates of vesicle release, the site usage tends to move from the center to the periphery of the active zone.
“Neurons often fire at 50 to 100 times per second, so it makes sense to have multiple sites,” Klyachko says. “If one site has just been used, the active zone can still be transmitting signals through its other sites.
“We’re studying the most basic machinery of the brain,” he says. “Our data suggest these machines are extremely fine-tuned—even subtle modulations may lead to disease. But before we can study disease, we need to understand how healthy synapses work.”
The Esther A. & Joseph Klingenstein Fund, the Whitehall Foundation, and the McDonnell Center at Washington University supported the work.