Under the right conditions, ordinary clear water droplets on a transparent surface can produce brilliant colors without any inks or dyes.
In a paper in Nature, a team of researchers describes how a surface covered in a fine mist of transparent droplets and lit with a single white light lamp can produce bright, iridescent colors if each tiny droplet is precisely the same size. This form of structural color could aid in the development of brilliantly colored cosmetics, color-changing paints, or adaptive camouflage.
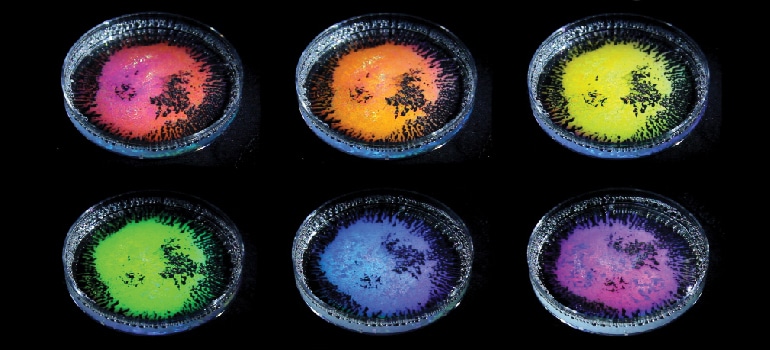
“The typical way you get color is with dyes or pigments, which have molecules that selectively absorb and scatter specific wavelengths of light,” says Lauren Zarzar, assistant professor of chemistry at Penn State and a leader of the research team.
“Structural color is different. It’s a product of light interacting with a material in a way that causes light interference. Structural color is often iridescent and the color we see depends on the angle we are looking from and the angle of the light. We see it in things like opals, butterfly wings, beetles, and bird feathers.”
Surprising colors in the petri dish
The team first noticed the phenomenon while studying transparent droplet emulsions made from a mixture of oils of different density and water-based surfactants—compounds that reduce surface tension in liquids and are used in soaps and detergents.
“Initially we followed the effect that causes rainbows, but it turned out to be something quite different.”
They were observing the droplets’ interactions in a clear petri dish, when they noticed the drops appeared surprisingly colorful, and the colors changed with viewing direction. Subsequently, the team was able to produce the same color effect by simply allowing water droplets to condense on the lid of a petri dish filled with warm water.
“We didn’t set out to discover this,” says Amy Goodling, a graduate student in materials science and engineering at Penn State and co-first author of the paper. “We have been working with these droplets for a while because the combination of oils and surfactants create droplets with a particular internal structure. It wasn’t until we started making droplets that were uniform in size that the color became noticeable.”
It’s not like rainbows
Where does the color come from? Initially, the researchers thought the color might be caused by the same phenomenon that produces rainbows. Rainbows form when sunlight enters a spherical raindrop and is bent, or refracted, off the back of the raindrop, before reflecting back out at a different angle.
“Initially we followed the effect that causes rainbows,” says Sara Nagelberg, a graduate student at MIT who led the modeling effort to try to explain the effect. “But it turned out to be something quite different.”
The droplets that produced color are not perfect spheres like those that form rainbows. Instead, both droplets of water that condense on a surface and the internal structure of the specialized oil and surfactant droplets have a hemispheric—or dome-like—shape. Light behaves differently in hemispheres than it does in spheres. Specifically, the curve of the hemisphere and the sharp change in refractive index—a measure of how fast light passes through an object—between the inside of the hemisphere and outside of it allows an optical effect that is not possible in perfect spheres: “total internal reflection.”
“…companies are asking, can we use structural colors to replace potentially unhealthy dyes?”
Total internal reflection occurs when light hits a boundary between substances with different refractive indexes at a specific angle such that one hundred percent of the light is reflected, rather than losing some of the light to refraction. By contrast, rain droplets that form rainbows only reflect about five percent of the light that enters them. The rest of the light is transmitted.
Once light makes its way into one of the hemispherical droplets, the researchers found that it can take different paths, bouncing two, three, or more times before exiting at another angle. The way light rays that have taken different paths through the droplet add up and interfere with each other as they exit determines whether a droplet will produce color or not.
“It’s like kids making waves in a pool,” says Mathias Kolle, assistant professor of mechanical engineering at MIT and an author of the paper. “If they do whatever they want, there’s no constructive adding up of effort, and just a lot of mess in the pool, or low random wave patterns. But if they all push and pull together, you get a big wave. It’s the same here: If you get waves in phase coming out for a particular color, that color is more intense.”
Replace dangerous dyes?
The researchers developed a model that predicts the color a droplet will produce given specific structural and optical conditions, such as the size and curvature of the droplets, along with the droplet’s total internal reflection.
“What’s really cool is that anyone can produce this affect with a few simple kitchen supplies.”
Nagelberg and Kolle incorporated all these parameters into a mathematical model to predict the colors that droplets would produce. Zarzar and Goodling then tested the model’s predictions against actual droplets they produced in the lab. The model could be a design guide to produce, for example, droplet-based litmus tests, or color-changing powders and inks in makeup products, paints, or displays.
“Synthetic dyes used in consumer products to create bright colors might not be as healthy as they should be,” says Kolle. “As some of these dyes are more strongly regulated, companies are asking, can we use structural colors to replace potentially unhealthy dyes? Thanks to the careful observations by Amy and Lauren at Penn State and Sara’s modeling, which brought this effect and its physical explanation to light, there might be an answer.”
Droplets with precisely controlled sizes lit from a single light source produce brilliant colors that shift from blue to green and yellow and red as you move around them. Changing the size and shape of the droplets changes the colors they produce.
“What’s really cool is that anyone can produce this affect with a few simple kitchen supplies,” says Zarzar. “In fact, you probably have already seen it when stretching plastic wrap over a container of warm food, or on the lid of a clear plastic container. It’s an everyday experience, not a phenomenon that requires complex lab equipment to create, it’s just that until now, no one had thought to try to explain it.”
In addition to liquid droplets, the researchers 3D-printed tiny, solid caps and domes from various transparent, polymer-based materials, and observed a similar colorful effect in these solid particles, which the team’s model could predict.
The research team expects that the model may be used to design droplets and particles for an array of color-changing applications. “There’s a complex parameter space you can play with,” Kolle says. “You can tailor a droplet’s size, morphology, and observation conditions to create the color you want.”
Additional collaborators are from Penn State and Sandia National Laboratories in Albuquerque, New Mexico. Support for the work came from Penn State; the US Army Research Office through the Institute for Soldier Nanotechnologies at MIT; the US National Science Foundation; and the Thomas and June Beaver Fellowship.
Source: Penn State