In a mine where workers once risked their lives to find gold, researchers now seek the ultimate treasure in particle physics—dark matter.
The Cage, as the elevator is called, leaves exactly at 7:30 am and slowly descends. Nearly two dozen people packed together inside wear coveralls, hard hats, and thick rubber boots to protect them from the water dripping from the timber buttressing the elevator shaft. (It’s kept wet to prevent them from drying out and rotting.) About 10 minutes later, nearly one mile down, the Cage thumps to a stop and the heavy, yellow metal doors swing open.
The men and women stream into a cavern with rough rock walls that miners once blasted with explosives in search of gold. Underfoot run the rail tracks used to wheel minecarts laden with equipment and supplies to the surface.
Further down one of the corridors, it starts to feel more civilized. Concrete walls and desks line the hallway, and fluorescent lights guide the way. There’s also a table with an espresso machine and panini maker.
Anyone venturing further must pass through a clean room where you remove your overalls, don a double layer of disposable booties, and have your possessions swabbed with alcohol.
A bit further and double doors swing open to reveal a lab. Scientists adjust equipment and take measurements. But the real center of attraction is a hulking 26-foot by 20-foot stainless steel vat in the middle of the room. In late March, the members of Brandeis University assistant professor of physics Bjoern Penning’s lab were inside it, hard at work.
Welcome to SURF
Until 2002, it was a working mine in the small town of Lead, South Dakota. Then, with state, federal, and private funding, it became the Sanford Underground Research Facility (SURF). Now, Penning and his team use the space to seek dark matter.
A mysterious, elusive substance, dark matter is thought to comprise around 85 percent of all the matter in the universe. The stuff we’re familiar with, atoms, makes up only 15 percent. We’ve known about protons, neutrons, and electrons for over 100 years, but we know next to nothing about dark matter. Evidence of its existence is overwhelming. Only by factoring in the extra mass it provides can scientists account for gravity’s effect on the arrangement and motion of galaxies.
But to date, no one’s been able to observe or detect dark matter. So Penning and his team have come to SURF to find it.
They are part of an international consortium of over 250 researchers worldwide working on what’s called the LUX-ZEPLIN (LZ) experiment. If LZ scientists succeed, it will be a revolutionary discovery that will give us a new understanding of what the cosmos is made of and how it came to be.
Penning and four lab members—senior mechanical engineer Andrei Dushkin, electrical engineer Richard Studley, graduate student Luke Korley, and postdoctoral fellow Ryan Wang—came to South Dakota to help build a dark matter detector.
Finding WIMPS
The stainless steel cauldron in which they worked is its outer shell. Dark matter is most likely made of WIMPS, weakly interacting massive particles. WIMPS are all around us, but because they’re so weak, they barely interact with other matter. This makes them “ghost particles”—they can pass right through us without registering any discernible impact.
WIMPS emit such a faint signal that they’re extremely hard to discern from all the other matter around us. The dark matter detector is designed to filter out all this background noise.
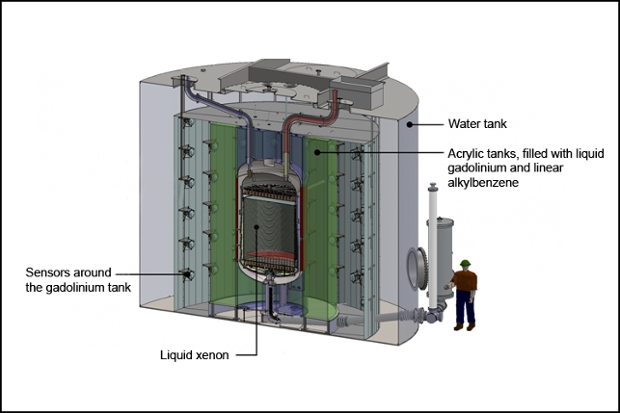
The detector functions like a set of nested sieves, each one filtering out other particles so that what finally winds up in the center can only be a WIMP. Penning likes to invoke a Sherlock Holmes’ quote he’s slightly amended—”When you have eliminated all possibilities, whatever remains, however improbable, must be the truth,” the truth, in this case, being a WIMP.
The outermost sieve is actually the mountain in which the lab is buried. The dirt and rock block out by a factor of a billion the cosmic radiation particles in our atmosphere left over from the Big Bang.
Next comes the 60,000 gallons of water that will be poured into the stainless steel container. It will be ultrapure, free of contaminants that might make dark matter hard to detect, and capable of screening out gamma radiation and neutrons.
Neutrons are a particular problem since they induce a weak signal that can easily be mistaken for WIMPS. Therefore, a second sieve was added to block neutrons—10 12-foot acrylic tanks suspended in the ultrapure water and filled with liquified gadolinium and linear alkylbenzene, a common component of cleaning products. Gadolinium atoms are arranged in such a way that neutrons stick to them.
Penning’s team designed sensors to surround the acrylic tanks. At present, they are non-working test dummies made on a 3D printer and look like giant white K-Cups. They will hang on scaffolding erected along the stainless steel tank’s perimeter.
When neutrons collide with gadolinium atoms, photons are emitted. The sensors can detect these photons, ensuring everything is working as planned and no neutrons are slipping through the gadolinium barrier.
The innermost sanctum of the LZ experiment—and its pièce de résistance—is a 13-foot titanium cylinder filled with liquid xenon. It will be submerged in the ultrapure water and encircled by the acrylic tanks.
If scientists’ predictions about the behavior and composition of WIMPS are correct, when one bumps into a xenon atom, it will produce two flashes of light that only dark matter could generate. Sensors inside the titanium cylinder will probe for this signature WIMP fingerprint.
Like a ship in a bottle
During their time in South Dakota, Penning’s lab only practiced setting up the sensors they’ve built for the detector. Everything will need to be kept ultra-sterile inside the detector when they do the final installation. The team will need to be extra careful not to contaminate the area.
A speck of dust inside can create enough background noise in the form of subatomic particles that drown out a WIMP signal. The crew will have to wear white coveralls and booties to prevent contamination. Even a dropped tool risks embedding impurities in the floor.
There is another big challenge, as well. When they finally install the sensors, the titanium container and the acrylic tanks will already be set up inside the stainless steel vat. This will leave only a three-foot wide area around the detector’s inside perimeter for them to work in. They will build the scaffolding for the sensors one piece at a time, moving around the perimeter. Finally, they will end at the small entrance and exit portal.
Penning and his crew will bring a ladder with them to erect the scaffolding. But when the scaffolding is completed, there won’t be enough room to maneuver the ladder. The ladder is 10 feet; the exit is a 4-foot wide portal. The only solution is a custom-made ladder that can be taken apart or folded into the size of a suitcase.
Penning likens the entire construction process to “building a ship in a bottle,” though in this instance they will be working from within the bottle.
If everything goes as planned, the whole contraption will become operational in 2020 and run for three years. Penning is guardedly optimistic. “Our experiment is the one with the best chance in the next few years if nature is kind,” Penning says. “But what nature does isn’t up to us. We just discover it.”
Source: Brandeis University