Physicists have opened the door to the first direct measurement of Berry curvature in solid matter—something of a holy grail among physicists.
A powerful unifying principle in several branches of classical and quantum physics, Berry curvature is a strange and elusive quantum mechanical property of solids. It governs the dynamics of the motion of charges in semiconductors yet itself cannot be directly measured.
If it could be, the resulting calculation could lead to new materials for quantum computing.
To navigate through an annotated 3D rendering of the experiment with virtual reality capability, click here.
The new work, described in the journal Physical Review X, builds on a previous paper in which the University of California, Santa Barbara researchers describe experiments resulting in an electron-hole recollision achieved by aiming high- and low-frequency laser beams at a semiconductor made of gallium arsenide.
For the new paper, scientists from UC Santa Barbara’s Sherwin Group collaborated with colleagues in China, at Princeton University, and at the US Naval Research Laboratory to improve on the previous experiment. They discovered a surprising new phenomenon using the same semiconductor subjected to extremely strong laser fields oscillating nearly 1 trillion times per second (1 terahertz). Called dynamical birefringence, scientists can use this phenomenon to investigate Berry curvature.
“When we originally did the experiment, we could only detect one sideband at a time and the samples were very fragile and more difficult to work with,” explains corresponding author Mark Sherwin, director of the Institute for Terahertz Science and Technology and a professor in the physics department.
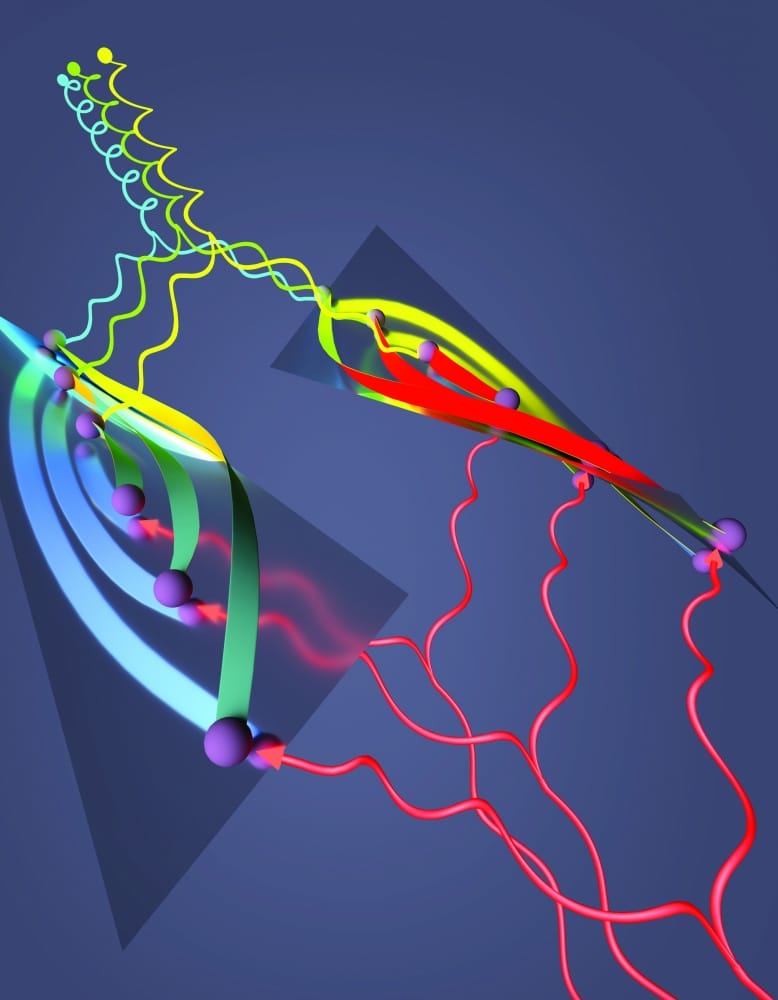
Experiment upgrades
Hunter Banks, lead author of the new paper, installed a camera that allowed the team to see all the sidebands simultaneously, which decreased the experiment’s duration and increased its sensitivity. He also improved how the samples were mounted and increased the strength of the terahertz electric field that the team could apply.
These enhancements revealed sidebands separated by as much as 90 times the photon energy of the terahertz—more than three times the amount in the original experiment. Sherwin notes that an increased quantity of sidebands allowed the team to learn more about the semiconductor. “As far as we know, this huge number of sidebands is the highest-order nonlinear optical process in solids,” he says.
Generated by a unique laser, these experiments drive thin layers of semiconductor while weak infrared light illuminates them. The infrared light is polarized in one of two ways: either parallel or perpendicular to the terahertz field.
Rainbowlike spectrum
“The infrared light transmitted through the semiconductor exhibits a rainbowlike spectrum containing dozens of frequencies, or sidebands,” Sherwin explains.
To create a better clock, apply this quantum ‘magic trick’
“Unexpectedly, the sidebands are usually stronger when the infrared beam is polarized perpendicular to the terahertz field. Somehow the terahertz is actually defining an axis that acts like a polarizing one. We call this phenomenon dynamical birefringence, and it arises as a direct consequence of Berry curvature.”
It also creates possibilities for applications in new classes of electronic and optical devices.
“We’re planning to turn dynamical birefringence into a direct measurement of Berry curvature,” Sherwin explains. “Once you can measure something that’s a basic property of a solid, then, when you’re designing new materials, you can optimize the Berry curvature for a particular device.”
Source: UC Santa Barbara