Researchers have successfully used laser cooling on radium ions for the first time.
Given that lasers are known for heating things up, laser cooling may seem a contradiction in terms. However, scientists have devised a way to use the technology to achieve unparalleled levels of cold.
Radium is the heaviest alkaline earth element, and the only ion in the column that hadn’t been laser cooled.
“I look at this as having the potential for discoveries as well as for constraining theories beyond the Standard Model of particle physics,” says Andrew Jayich, an assistant professor of physics at the University of California, Santa Barbara. Radium shows promise in diverse fields like quantum information science, optical clocks, and fundamental physics research.
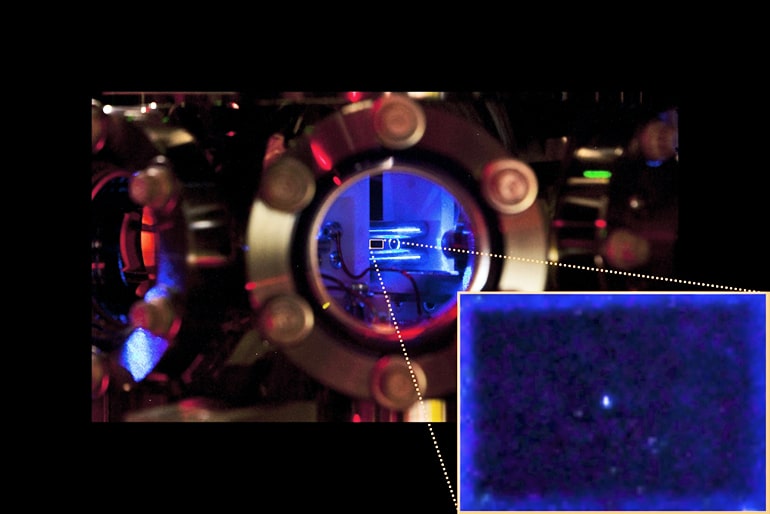
To achieve laser cooling, scientists start with a charged atom, an ion, that is trapped with electric fields. Imagine the hot atom bouncing around in an ion trap as a playground swing in motion. Give it a push at the right time and you’ll send it higher, or, in this analogy, heat up the atom. But a push opposing the swing’s motion, as it’s coming toward you, will actually slow it, which cools the atom.
Each energy state an atom can take has an associated resonance frequency. Scientists can coax the atom to transition into a specific state by hitting them with laser light tuned to that specific frequency. The atom will absorb the light and transition to the desired state. Then it will drop to a lower energy state, emitting its own photon that carries away some of the atom’s momentum. This literally slows the atom down, and a slow atom translates to a colder atom.
Tuning the laser slightly below this resonance frequency makes it more likely you’ll give the atom a push as it’s headed toward the beam, rather than away. It’s a result of the Doppler effect, which shifts the frequency up as the atom moves towards the incoming laser, much like the siren of a fire engine has a higher pitch as it approaches. Thanks to this method, scientists are never pushing the swing harder, only slowing it down.
Laser cooling and detecting a trapped ion is tricky, and the fact that radium has no stable isotopes made the first laser cooling even more challenging for the researchers. Because the element is radioactive, they initially had only 10 micrograms to work with, and were concerned they’d use up their sample before finding the correct parameters for trapping and cooling the ions. Finally, after several weeks of searching, the camera caught its first glimpse of a laser-cooled radium ion. This new paper represents nearly two years the team spent constructing ion-trapping equipment and perfecting their laser-cooling technique.
‘A nuclear amplifier for new physics’
For a long time, scientists thought that physics was symmetric—that it worked the same if you flipped the values of the charges or the orientations of all the particles in a system. They also assumed things played out the same going forward in time as backward. Surprisingly, experiments have shown that these basic assumptions about symmetry are not true. Only the three symmetries—charge, parity, and time—taken together appear to always hold up. Namely, every physical process we know of stays the same if you swap right for left, positive for negative, and future for past.
While physicists have identified processes that violate charge-parity symmetry in fundamental particles, the violations aren’t large enough to explain longstanding questions, like why the universe has more matter than antimatter.
“Theories beyond the Standard Model usually add in new charge-parity symmetry violations to explain these puzzles,” says lead author Mingyu Fan, a doctoral student in the Jayich lab. “The symmetry violations predicted by new physics models would also affect atoms,” and the heavy nuclei of radium atoms are particularly susceptible to displaying these behaviors. That’s why the team has devoted so much time and energy to learning how to manipulate this element.
The radium nucleus also has a unique shape, like a pear, and a set of two of nuclear states separated by only a small amount of energy. These features make radium more sensitive than other nuclei to time symmetry violation, physics that has not been observed in any atom or molecule, Jayich explains. Some yet undiscovered physics—like a new particle or interaction—might mix these two nuclear states.
Jayich suspects that radium will enhance sensitivity to symmetry violations by 1,000-fold compared to the best systems currently in use. “Radium is like a nuclear amplifier for new physics,” he says.
Future experiments
The team’s initial success in laser-cooling radium ions opens the door to an impressive set of experiments and applications that Jayich intends to pursue in the coming years. In terms of technology, a heavy atom like radium is less susceptible to getting bumped around, thereby minimizing noise in a trapped ion system.
Now the team has to actually measure these states. “Very basic properties of the radium ion, such as lifetimes and branching ratios, things that an atomic physicist would take for granted, have not been measured because it hadn’t been laser cooled,” says Jayich. “We need to measure the radium ion’s basic properties so we can build advanced experiments. We hope to finish these fundamental measurements within the next year.”
The team is planning a new experiment using a different form of radium with a nuclear spin that will open new physics opportunities. Although the radium-225 has a half-life of only 15 days, the team will use a clever method to continuously replenish their supplies using an isotope of thorium that radioactively decays to radium-225. The thorium isotope has a 7,300-year half-life, so the team isn’t concerned about running out. As a bonus, the two elements are very easy to separate, ensuring that no thorium contaminates the sample.
Laser cooling radium also opens up the possibility of putting the atom inside molecules. The group plans to make and study radium hydroxide in their ion trap, which is trickier than simply synthesizing a compound in a test tube. This would increase sensitivity to new physics by another factor of 1,000 compared to just an atomic system, Jayich says.
Time will tell. The team’s planned experiments in this line of research could take several years to yield results once they are up and running, he adds.
“It’s really the sum of many things that come together to make radium special,” Jayich says, “and they all come together to give you a really nice platform for both fundamental physics and photonic quantum technologies.”
The researchers’ findings appear in the journal Physical Review Letters.
Source: UC Santa Barbara